by Anthony Illingworth
Overview. In the last two decades the accuracy of weather forecasts has been transformed due to better
assimilation of observations and consequent improvement in the initial state of the forecast model’s
representation of the current weather. Bauer et al (2015) note that accurate forecasts save lives, support
emergency management and the mitigation of impacts thus preventing losses from high-impact weather,
while creating substantial revenue, for example, in energy, agriculture, transport and recreational sectors.
They comment that sparse observation of winds is an impediment to increased accuracy and suggest that
direct observations of winds by Doppler-radar technology could alleviate this problem. This promise has
been realised by the winds from ESA’s Aelous lidar mission launched in 2018 that are already reducing
errors in the ECMWF forecast model by more than any other single instrument (Rennie et al., 2020).
We propose a space-borne dual-polarisation Doppler conically scanning 94 GHz radar measuring incloud line-of-sight winds over an 800-km wide ground track using the returns from cloud and precipitation targets (see Figure 1) and provides winds with better than 2m/s accuracy over a 20km distance along the cycloidal ground track for echoes above -20dBZ. These in-cloud winds would complement the Aeolus observations and satisfy the WMO requirements for Numerical Weather Prediction (NWP) models of horizontal winds with 1 km vertical resolution and global coverage.
The PRIMARY OBJECTIVE of the WIVERN mission is to provide global measurements of in-cloud
line-of-sight winds with three major applications.
- Assimilation of the “large-scale” (> 20 km) in-cloud wind observations with quantified errors into
NWP models to further improve their forecast skill. Currently, measurements inside optically thick clouds
are sparse and only available from instrumented aircrafts taking off and landing. - Velocity fluctuations on the km scale should provide information on the characteristics of convective
motions. Representation of convection and the links between dynamics and precipitation are major
challenges requiring global observations. NWP and climate models use similar parameterisation schemes
so better NWP models (whose performance can be validated every day) will also improve climate models. - Unique observations of profiles of winds within tropical cyclones and hurricanes that are currently not
available operationally but are possible only from occasional penetrations by instrumented aircrafts.
The SECONDARY OBJECTIVE is to deliver global rainfall, snowfall and cloud ice water content from
the radar reflectivity of the hydrometeors extending the 14-year data set that has been provided by
CloudSat since 2006 but with at least a forty-fold increase in sample coverage. Assimilation of CloudSat
reflectivity data shows a small positive impact in reducing forecast error so WIVERN’s improved
sampling promises a much more significant error reduction. In addition, a sensitive 94-GHz brightness
temperature mode will be implemented to better detect supercooled clouds and large ice particles.
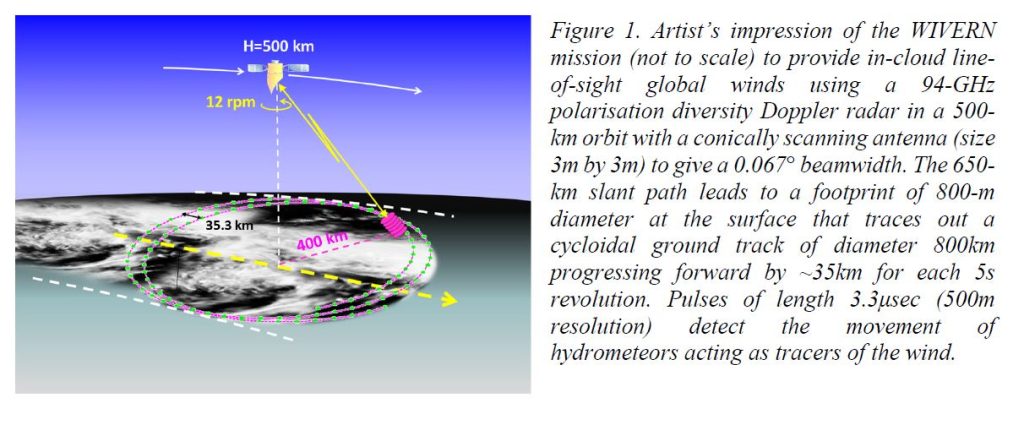
Heritage. WIVERN will use the legacy of the 94 GHz Extended Interaction Klystron (EIK) that CloudSat
has operated since the 2006 launch with the same 3.3 μs (500 m) pulses and a similar 4kHz Pulse
Repetition Frequency. WIVERN’s innovation will be to use polarisation diversity. The EIK power supply
will be based on recent pre-developments during SKIM EE9 phase A and for the METOP-C scatterometer.
Predicted Doppler Performance. Doppler measurements from a moving space platform are challenging.
ESA funded studies with ground-based and airborne 94 GHz radars have validated the polarization
diversity technique and the accuracy of the derived winds as a function of the signal to noise ratio (SNR)
of the return echo. It has been shown that any biases due to non-uniform beam filling or the presence of
high vertical shear are rare and can be corrected, and that any cross talk between H and V-polarised returns can be recognised and flagged. These findings are summarised by Illingworth et al. (2018) and other peerreviewed papers. The SNR of WIVERN can be derived by scaling the known performance of CloudSat for WIVERN’s 650-km slant range and larger antenna. For this SNR the WIVERN winds should be
accurate to 2m/s for target reflectivities > -20dBZ and 20km integration along the cycloidal ground track.
Finally, based on CloudSat’s global climatology of cloud reflectivities, WIVERN should provide about
one million winds per day accompanied by a quantified error estimate as is required for data assimilation.
WIVERN obtains line of sight (LOS) winds at 41.6° off zenith but the requirement is for the horizontal
component of the LOS wind (HLOS), so a knowledge of the convective up and downdraughts and the
terminal velocity of the hydrometeors is required. Analysing a large data set of high-resolution 3D winds
and reflectivity obtained from an airborne 94GHz Doppler radar, reveals that terminal velocity is a robust
function of the reflectivity and the temperature, and that stratiform regions can be identified by the small
variations in the km-to-km LOS winds over 20km. In such regions the HLOS retrieved velocity in ice
clouds agrees closely with the true horizontal wind speed measured directly by the airborne radar; such
winds with errors should be representative of the large-scale flow and be suitable for data assimilation.
Matched Beam 94GHz Brightness Temperature (Tb). A relative accuracy of 3-4K for each km along
the cycloidal track (with 0.1K stability over 100km) will be achieved by measuring the noise level of a
500 MHz bandwidth receiver channel during times when there are no atmospheric returns, rather than the 300 kHz bandwidth used by CloudSat and EarthCARE, to better detect liquid water and large ice particles.
Technical issues with the antenna and feed. The rotating antenna will induce a sinusoidal velocity with
a period of 5 s and an amplitude 5000m/s which can be removed if the antenna pointing is known to better than 200μrads (~ 0.01°). Recent industry studies of SKIM confirm that 1-μrad pointing knowledge can be achieved through the combined use of star trackers, a shaft encoder, a gyroscope and ground processing using ocean surfaces as calibration targets. The thermoelastic stress on the antenna should also meet a 20-30μrad specification. The elevation angle of the rotating antenna can be monitored by operating the radar in altimeter mode. A 200-μrad change in elevation angle leads to a change in range of the sea surface of over 100 m that can be easily detected. Different strategies for mitigating low frequency (e.g. orbital) pointing biases are also available, for example (as demonstrated by Aeolus), the winds on the left and right side of the satellite for ascending and descending orbits averaged over a day should be equal and opposite. An ESA study (Lori et al. 2017) has produced a design and tested a breadboard implementation to feed the H and V pulses to the rotating antenna.
SRL. Current SRL is 3 with a road map to level 5. An end-to end simulator has been developed and tested.
TRL. Industry has prepared a road map to achieve TRL5 by the end of phase B1.
Fit to ESA Priorities and International Aspects. The ESA science strategy (SP-1329/1) highlights (p8
– ‘disasters’) the need for Europe to cope better with storms and floods and (page 19) “the major challenge to forecast climate change by understanding the links between clouds, atmospheric circulation and precipitation”. The USA National Academy of Science (NASA 2018) decadal survey has recommended
six designated priorities, one of which is “Clouds, Convection and Precipitation” with a train of radars to
study process studies and convection. This would be complementary to the proposed WIVERN mission.