Lead Organisation: Durham University
Project Lead: Cyril Bourgenot
Durham University has developed a new instrument concept, based on technologies imported from astronomical instrumentation, to improve and miniaturise hyperspectral imagers. The technology is based on the use of (i) image slicers (offering an extra dimension in the build-up of hyperspectral imaging data to deliver a large number of simultaneous contiguous spatial samples, together with reasonable coverage of the spectral domain) and (ii) freeform gratings, where a diffraction grating has been machined on top of a freeform surface to optimise compactness and instrument throughput. The freeform grating both reimages and disperses the light. This modality enables a more compact and lightweight instrument (critical parameters for UAV systems). Secondly, it targets specific spectral regions of interest at the required resolution, to dramatically improve data reduction and therefore ease the data transfer and storage.
A diagram of a multi-channel EO imaging spectrograph using integrated freeform gratings is shown below. As the scene moves over the image slicer mirror, each slice receives the same part of the scene sequentially (with a temporal delay due to satellite motion) and redirects the light towards a freeform grating. This powered grating is optimised to reimage the spatial content, and disperse the spectral content, directly onto a detector with minimal geometric aberrations. The arrangement of the gratings in a compact packed array is optimised to reformat the image slicer into a virtual pseudo slit. Each grating will therefore probe a specific spectral domain, at a defined spectral resolution, while the spatial resolution remains the same for all the channels. However, there are several issues which need to be addressed before these pioneering optical elements are brought to the next technology readiness level where the technology can be validated in a prototype instrument. This project has enabled the determination of the most suitable materials, as well as the machining parameters to produced high quality surfaces by ultra-precision diamond machining. The project has demonstrated that in theory, freeform gratings in the form of concave tilted off-axis ellipses are well suited for instruments with medium resolving power R < 10 000, with a spectral band dependant on the resolving power. Low or high order (up to order 6) can be used. Similar spectral resolution to the Orbiting Carbon Observatory (OCO) satellite can be achieved with this type of design in a much more compact instrument, but with a restricted bandwidth. Typically, low diffraction orders (m=1 or 2) are compatible with a bandwidth in the range of 20% to 70% of the central wavelength in order to keep the optical quality below the diffraction limit. Higher orders (m =4, 5 or 6) will have a restricted bandwidth in the range of 1% to 5% of the central wavelength in order to maintain good optical quality.
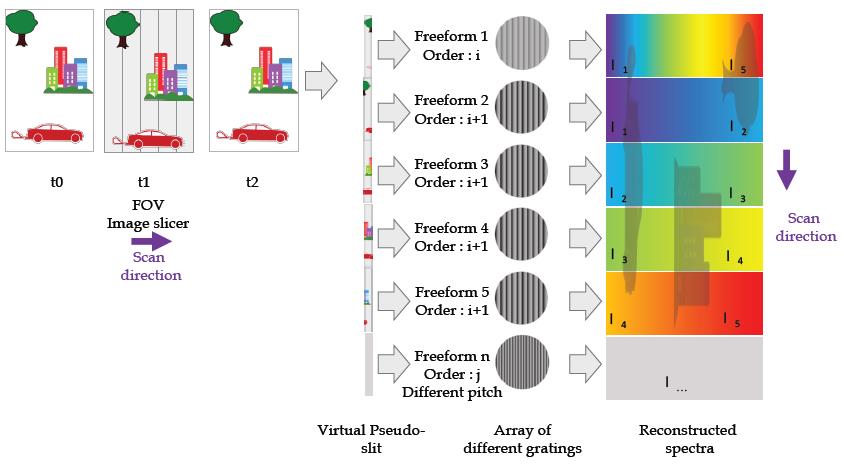
In practice, the best optical performances were obtained by diamond machining (ruling method) on a nickel substrate, with a typical roughness of about 2-3 nm RMS. The optical performance of these gratings was found to be limited by the form error that is created during the long machining processes (about 30 hours for a 50mm diameter grating with a groove period of 10 microns). This form error is believed to be the result of thermal variations inside the machine structure. The form error is worse along the direction normal to the grating lines, and this could affect the spectral resolution more than the spatial resolution. With thermal stability improved, diamond machined metallic freeform gratings can offer a viable solution for remote sensing spectrographs on an airborne or space platform. Greenhouse gas monitoring can benefit from the compactness and versatility of this technology. A system similar to the Orbiting Carbon Observatory could be implemented on nanosatellites for example to target narrow spectral bandwidth at mid to high spectral resolution.
This technology has the potential to overcome some of the limitations of conventional pushbroom hyperspectral imagers including: (i) most pushbroom scan systems use a single dispersive element which restricts either the waveband coverage or the spectral resolution; (ii) most pushbroom systems incorporate multiples optical elements, increasing mass and cost. The new capability investigated in this CEOI Pathfinder project would allow multiple wavebands and spectral resolutions to be imaged on a single detector, significantly simplifying the system while maximising the throughput.